Inno4scale is a European initiative, which supports the development of innovative algorithms for exascale supercomputers, so their efficient use can be fully exploited. Currently existing codes for high-performance computing will not be able to function efficiently on upcoming exascale systems in the future. Therefore, the project will identify and support the development of applications that have the potential to fully exploit the new upcoming EuroHPC exascale systems. CEM investigates with KU Leuven parallel-in-time methods.
Magnetocaloric cooling technology has the potential to increase energy efficiency by 40% compared to conventional gas-steam compressor cooling technology and also eliminate all direct greenhouse gas emissions. This could replace air conditioning systems in supermarkets, offices, or industrial plants that today still rely on climate-damaging refrigerants. MAGNOTHERM is working on the commercial introduction of the novel magnetic cooling technology, which exploits the magnetocaloric effect of certain materials, i.e. the temperature change associated with a magnetic field change. In the LOEWE project OptiMag, the magnetic source is to be optimised in terms of its shape and the distribution of soft iron, permanent magnets and magnetocaloric material in order to increase efficiency and reduce costs, thus decisively advancing the market maturity of magnetic cooling. This a collaborative project with the cyber-physical simulation group.
TU Darmstadt, TU Graz and JKU/RICAM, Linz, investigate how electrical machines can be decisively improved through computer simulation. To this end, the first German-Austrian Collaborative Research Centre/Transregio – TRR361/F90 has been approved. The German Research Foundation (DFG) and the Austrian Science Fund FWF are funding the TRR “Computational Electrical Machine Laboratory” with a total of over eight million euros. The project s coordinated be CEM.
In all lighting sectors, warranty and customisation are becoming key product differentiators. In addition to that, the integration of more electronics and sensors in lighting systems will change what we call lighting today. While the concepts of digitalisation and Industry 4.0 are progressing fast into the manufacturing world, in the lighting industry, the front-end product design is still using traditional simulation techniques. An innovative approach is to couple digital twins with Artificial Intelligence to offer unlimited possibilities to the “first build and then tweak” approach. The main goal of AI-TWILIGHT is to merge the virtual and physical worlds to pave the way for innovations in fields where the European lighting industry is likely to be competitive. Self-leaning digital twins of lighting systems (LED source, driver of a lighting application) will be created and used as input for predicting performance and lifetime of product and infrastructure design and management in an autonomous world. Tests will be carried out in selected application domains e.g. automotive, horticulture, general and street lighting.
The software Bembel is supported by the German Research Foundation in the program for sustainable usability of research software. The goal of the program is to reuse and further enhance research software that has the potential to be used in research significantly beyond its original context, and to make it accessible for quality assurance by an expert community. The project is oriented towards best practice examples for user-centered further development and community-based quality assurance.
The project Time-X will develop new, parallelizable algorithms for time-dependent simulations, which so far could not be performed in a reasonable time frame due to their immense computational effort. These algorithms allow parallelization along the time dimension for time-dependent simulations and these are provided in software libraries. This is a necessary step to efficiently use the computing power of future high-performance computers in and beyond the exascale range for model-based predictions. Due to the ever increasing demand for simulations, especially in industrial applications, these new algorithms will also play an important role in the economy.
NHR4CES (National High Performance Computing Center for Computational Engineering Sciences) is part of the association for National High Performance Computing (NHR). NHR aims to provide scientists at German universities with the computing capacity they need for their research, and strengthen their skills for the efficient use of this resource.
In NHR4CES, RWTH Aachen University and Technical University Darmstadt join forces to combine their strengths in HPC applications, algorithms and methods, and the efficient use of HPC hardware. Our goal is to create an ecosystem combining best practices of HPC and research data management to address questions that are of central importance for technical developments in economy and society.
This project proposes the application of a Spline or more precisely NURBS-based finite element approach for the simulation of electrical machines, the so-called isogeometric analysis. This novel approach promises accurate solutions with a low number of degrees of freedom and enables elegantly to model geometry variations due to the Spline parametrisation, e.g. within an uncertainty quantification or shape optimisation of a machine. The movement of the rotor is implemented by mortaring in a saddle-point formulation. We propose novel approaches based on Splines and spectral elements which will be analyzed theoretically and practically.
Foil windings are increasingly applied in electrical energy converter because of their high fill factor, low DC resistance and advantageous thermal properties. They, however, feature a specific eddy-current effect related to the uni-directional redistribution of the currents towards the foil tips, and a comparably large interturn capacitance, related due to the thin insulation layers. Today, a sufficiently accurate simulation of these effects is only possible with three-dimensional finite-element models in which all geometric details in the foil-winding parts are resolved. The unmanageable size of the overall models impedes a further improvement of foil windings in contemporary electrotechnical designs. The outcome of this research project will be a finite-element foil-winding model which is capable of simulating eddy-current, capacitive and thermal effects in foil windings at a computational cost which is comparable to the one for standard wire windings. This goal will be achieved by an appropriate combination of analytical and computational homogenisation methods, a dedicated hybrid finite-element, spectral-element discretisation, error-controlled mesh adaptation, field-circuit coupling and multi-rate time integration. The performance and reliability of the developed numerical schemes will be demonstrated for machine foil windings, for a high-frequency foil-winding transformer and for a metallic film capacitor.
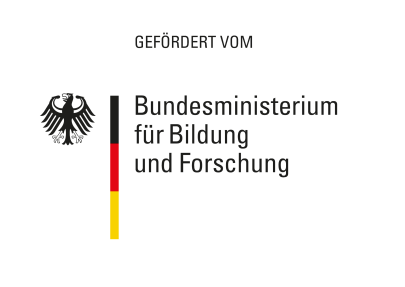
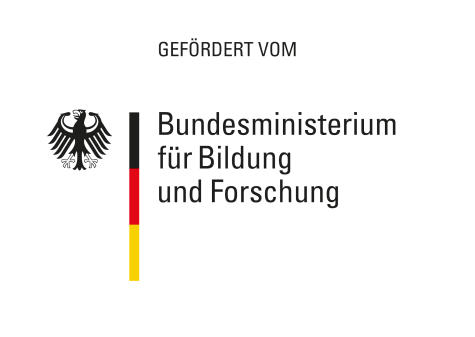
In order to achieve the required intensities and energies at new and particle accelerators under construction in the future, new and better diagnostic equipment and methods are needed. The increasing requirements can only be met with significantly improved devices, new measuring principles and a deeper understanding of the physical processes. These include principles for measuring the smallest particle streams, measuring the shortest particle packages, pressure measurement in cryogenic structures, beam profile measurements with as little impact and/or cost as possible, and reliable detection of quenches optimized for plant availability. In all subprojects, the challenges include increasing measurement accuracy and reliability of diagnostics, noise suppression, expanding application range, reducing investment and operating costs, and improving machine availability.
In the DIAGNOSE project, particle beam diagnostics and the monitoring of accelerator components are being further developed and in some cases newly developed, especially at FAIR (GSI) and HL-LHC (CERN). The upcoming work is of decisive importance for the efficient, high-performance and reliable operation of these research facilities.
The research project continues the previous work of the applicants funded by the DFG grants CL1431/1-1 and SCHO1562/1-1. The aim of this research project is to develop numerically efficient parallel algorithms for the calculation of eddy current problems, which are particularly suitable for current heterogeneous, massively parallel computer architectures. Since conventional implicit and recently developed (semi-)explicit time integration methods for magnetoquasistatic problems no longer scale well after a certain number of computing cores, time-parallel algorithms such as multiple-shooting methods or parareal methods are also used in this research project. These open up new parallelization potential along the time axis.
PASIROM is a collaborative project funded by the Federal Ministry of Education and Research (BMBF) in the program for the advancement of research in the field of mathematics for innovation. It builds on the results of the SIMUROM network (funding period 2013-2016). The research network investigates questions that are oriented towards the fields of demand of the German government's High-Tech Strategy 2020: Mobility, climate and energy. Efficient design of electrical or electromechanical energy converters, such as motors, generators and eddy current brakes, must take many factors into account. In simulations, systems with millions of unknowns must be solved. Multiphysical effects such as eddy currents, stimulation of electrical networks, rotor movements or heat generation must also be taken into account by the developers, so that high-resolution simulations of complicated devices often take a week or longer at present. And despite the highest possible accuracy, simulation results are often only rough approximations to reality. In order to avoid that small deviations from the reference design, for example in production, lead to unexpected reduced performance or failures, an oversizing based on empirical knowledge is necessary today.
NFDI4Ing brings together the engineering communities and fosters the management of engineering research data. The consortium represents engineers from all walks of the profession. It offers a unique method-oriented and user-centred approach in order to make engineering research data FAIR – findable, accessible, interoperable, and re-usable.
NFDI4Ing has been founded in 2017. The consortium has actively engaged engineers across all five engineering research areas of the DFG classification. Leading figures have teamed up with experienced infrastructure providers. As one important step, NFDI4Ing has taken on the task of structuring the wealth of concrete needs in research data management.
SIMUROM is a joint project funded by the Federal Ministry of Education and Research (BMBF) in the programme for the advancement of research in the field of “Mathematics for Innovations in Industry and Services” (Project Management Organisation: DESY, Hamburg). The goals of the collaborative project are problem-specific modelling and analysis as well as general method development that takes uncertainties into account and enables robust simulations. On this basis, parametric reduced models are constructed, which enable a robust optimization.
Drops play a central role in many areas of nature and technology. Examples from nature are rain, clouds or fog. With regard to technical processes, the evaporation of droplets in fuel sprays during combustion in vehicle engines or aircraft engines can be mentioned as an application, whereby these processes are directly linked to the formation of pollutants. The basic understanding of drip dynamic processes is crucial for the prediction of natural processes and the optimization of technical systems. Many of these processes take place under extreme environmental conditions, e.g. high pressure or extreme temperatures, and are already applied in technology, although there are still large gaps in basic understanding. This is exactly where the Transregio comes in. The aim is to gain a deeper physical understanding of the essential processes. Based on this, ways to analytical and numerical description shall be pointed out and these shall of course be implemented. In addition, the prediction of larger systems in nature or in technical facilities will be improved.
To calculate the resonant frequencies of superconducting cavity resonators for particle accelerators one needs numerical methods capable of achieving accuracies which push established techniques to their limit. This is mostly due to the precision the geometry description. It is described with Non-Uniform Rational B-Splines (NURBS), which can, contrary to the usual triangulations, describe the geometry exactly. This method became popular within the framework of Isogeometric Analysis (IGA) and has proven itself already in the context of finite element methods. Since the creation of a volumetric representation of this kind takes a lot of manual effort, and the boundary data of the required format is already given by CAD systems, the isogeometric approach shall be combined with a Boundary Element Method (BEM). Thanks to modern compression techniques and preconditioning, BEM is a viable alternative to a finite element method. Eventually, the algebraic eigenvalue problems generated by the boundary element method are nonlinear and contour integral methods will be used for their solution.
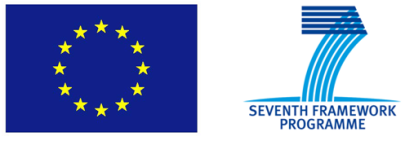
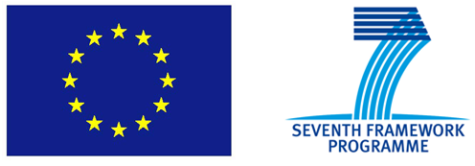
Designs in nanoelectronics often lead to problems that are large to simulate and that include strong feedback couplings. Industry demands to include variability to guarantee quality and yield. It also requests to incorporate higher abstraction levels to allow for system simulation in order to shorten design cycles, while preserving accuracy. Solutions are, advanced co-simulation/multirate/monolithic techniques, combined with envelope/wavelet approaches; generalized techniques from Uncertainty Quantification (UQ) for coupled problems, tuned to the statistical demands from manufacturability; enhanced, parameterized Model Order Reduction techniques for coupled problems and for UQ. All algorithms will be validated in the industrial design tools provided by our industrial partners. The consortium includes five universities, one research institute, two large-scale semiconductor companies, and three SMEs.
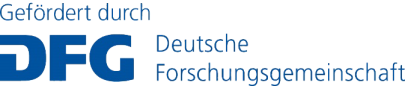
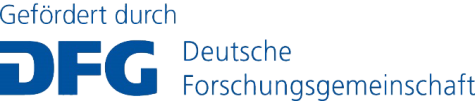
In this research proposal we will develop the fundamentals for new numerical methods and improve existing schemes for the efficient explicit computation of low-frequent electromagnetic fields. The aim is to solve larger problems in less time by using parallel computing architectures. We propose in particular the combination of Discontinous Galerkin Finite Elements with explicit Runge-Kutta time-integration methods. This allows to make good use of the computing power of multi-core architectures (e.g. general purpose graphics processor units) because many (parallel) operations can be performed with low data communication.
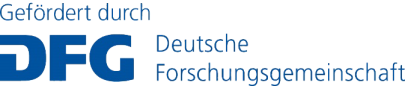
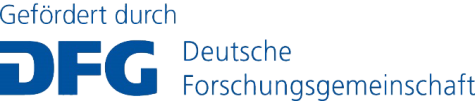
The scientific network Uncertainty quantification techniques and stochastic models for superconducting radio frequency cavities deals with the modelling and determination of uncertainties in the stochastic parameters and outputs of superconducting radio frequency resonators. The aim of the network is to develop and exchange models already used by the network partners to describe the input uncertainties (e.g. geometry parameters) as well as methods to determine the uncertainties in the outputs (e.g. eigenmodes) for a suitable benchmark geometry. These methods comprise deterministic, stochastic and composite approaches and are different efficient in their application depending on the problem. In the course of comparing the stochastic methods, the discretization methods used by the various network partners are also evaluated. The members of the scientific network plan to publish the jointly achieved results in a publication. The long-term goal is to integrate the stochastic methods into the optimization process for future resonator designs and thus to determine robust designs.
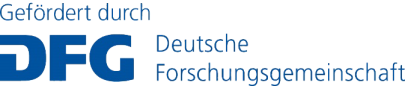
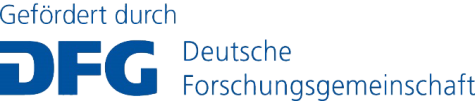
The intention of the CoSiMOR network is to provide an environment for the interdisciplinary exchange of advanced computational methods and possible fields of application. The focus is on multi-scale problems, e.g., in the sense of different time or length scales. From a methodological point of view, methods belonging to the class of co-simulation (CoSi) and model order-reduction (MOR) are investigated by the members of the network. Examples for such problems are found in electronic circuit simulation, where individual devices are replaced by fine resolution finite element models usually living at different time-scales. A possible solution technique for this problem class is co-simulation.